Advancing Widefield Calcium Imaging with LED Illumination
LED illumination technology has replaced traditional lamps for calcium imaging applications, and there are now many options available with a variety of different features.
Meanwhile, new calcium indicators and genetically encoded calcium indicators are becoming available across the spectrum, and it’s crucial to match the LED illumination system to the calcium indicator and other application requirements.
Find out:
- Why LED illumination is popular for calcium imaging
- Compatibility between LED illumination and popular calcium indicators
- How to choose the right LED illumination for Fura-2 imaging
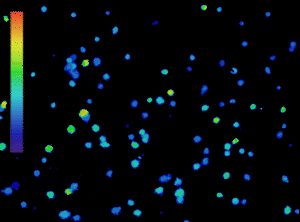
The importance of calcium in physiological processes needs no introduction. Utilising fluorescent calcium indicators to track, analyse and quantify calcium signalling under the microscope is a powerful tool for scientists (Figure 1), and technology in this area is continually evolving.
As new indicators emerge and light sources become more sophisticated, understanding the best setup to use can be challenging.
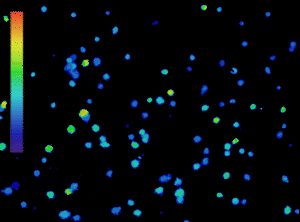
Figure 1: Calcium imaging is a powerful tool. Ratiometric imaging of lymphocytes labelled with Fura-2 obtained with the CoolLED pE-800fura for live calcium monitoring. Microscopy system equipped with Inscoper liveRATIO
Why are LEDs popular for calcium imaging?
The solid-state nature of LED illumination delivers numerous benefits when compared to traditional calcium imaging light source methods. These features include:
High-speed LED switching: Speeds of under 7 µs are possible with LED illumination. This increases temporal resolution for capturing extra detail for dynamic events, while also increasing the accuracy of ratiometric measurements. Moreover, powerful illumination enables the shorter camera exposure times necessary for this speed. The highest speeds are achieved with TTL triggering, which bypasses the latency associated with USB operation. Illumination can be tightly controlled via hardware, allowing integration into setups that might even combine calcium imaging with other applications such as optogenetics and electrophysiology.
Individual channel control: Unlike white light sources, LED illumination systems for calcium imaging feature discrete LED spectra which can be individually selected to match the indicator in use. Optical filters fail to completely block all unwanted wavelengths of a white light source, and therefore utilising the matching wavelengths increases the signal-to-noise ratio. This provides cleaner images and data whilst requiring less dye, reducing toxicity and cost.
Alongside these performance advantages, convenience is a factor in every laboratory. LEDs are not only simple to modulate with fast on/off and 0-100% intensity control, but also have a long lifetime, avoiding the requirement to change and re-align the bulb. And their environmental credentials are becoming an increasingly significant factor, being energy efficient while avoiding the use of toxic mercury.
Matching the calcium indicator and LED channel
Many calcium indicators are available, encompassing both chemical dyes and genetically encoded calcium indicators (GECIs). A multitude of factors must be considered when choosing the best indicator for an experimental setup, which are explained in more detail in this article from Andor. One of the most significant factors we will focus on here relates to the illumination method of choice.
Each indicator has its own spectral profile, the absorbance profile of which must match both the spectral output of the light source, and excitation filter. The simplest way to determine the compatibility is to use a spectral viewer such as FPbase.1 LED spectra can vary greatly in width, and understanding compatibility is not possible when considering an LED’s name alone. For example, GCaMP6f has a peak absorbance at approximately 500 nm, but it can be efficiently excited by the powerful 470 nm LED (Figure 1A). The nature of LED spectra allows coverage of calcium indicators outside the centre wavelength of the LED, and a few examples of these are shown in Figure 1B.
Figure 1: LED illumination coverage of calcium imaging dyes and GECIs. Unlike lasers, individual LEDs have wider spectra, allowing coverage of calcium indicators outside the centre wavelength. A) GCaMP can be imaged using an LED with a centre wavelength of 470 nm standard GFP filters, owing to an adequate overlap of spectra and powerful illumination (red). B) A selection of popular calcium dyes and GECIs and their compatibility with two LED Illumination Systems designed for calcium imaging. CoolLED also recommends optical filters on the filter finder tool, but the filter names often relate to common fluorophores, which are noted here for each calcium indicator.
There is also a trend towards red-shifted dyes which reduce phototoxicity and therefore lead to more accurate experimental results.
More complex experiments requiring spectral separation benefit from this development, which allows simultaneous imaging with fluorophores such as GFP, or optogenetic stimulation with ChR2.
Since LED Illumination Systems range up to a centre wavelength of 770 nm (the CoolLED pE-4000), there are many future opportunities to implement calcium indicators across a broad spectrum.
Fura-2 Focus
For quantitative experiments, ratiometric calcium indicators are the go-to approach, reducing effects such as uneven dye loading which can reduce measurement precision. Fura-2 is the most popular ratiometric dye, and requires excitation at 340 nm and 380 nm, which was not always possible with LED illumination. However, since CoolLED introduced the pE-340fura, LED illumination has become the mainstream solution.2 Increasing the accuracy of Fura-2 measurements, high-speed switching between the 340 nm and 380 nm LEDs is achieved through:
- Fast TTL switching (20 μs with the CoolLED pE-340fura and <7 μs with the pE-800fura)
- High power enabling fast image acquisition
- Ability to fit inline single-band excitation filters in front of the 340 and 380 nm LEDs removes the latency of a filter wheel
Figure 3: Fura-2 calcium imaging with the pE-340fura. Cardiac myocytes loaded with Fura-2 using standard conditions (i.e. incubation with 2 micromolar Fura-2 acetoxymethyl ester for 30 minutes, followed by an additional 30 minutes for de-esterification. (Videos acquired by Martin Bootman and Katja Rietdorf). The left-hand and right-hand videos show the Fura-2-loaded cells with 340 nm and 380 nm excitation, respectively.
LED Illumination Systems for Fura-2
The CoolLED pE-800fura includes eight individual LED channels, and is designed to suit a range of applications in addition to Fura-2 imaging, from pH monitoring and optogenetics to everyday fluorescence microscopy. Moreover, the industry-leading <7 µs LED switching speeds maximise temporal resolution, capturing extra details during high-speed experiments.
This is ideal for research labs demanding high-quality data but where it isn’t feasible to dedicate an entire microscope setup to Fura-2 imaging. Instead, a single widefield microscope can be used for a multitude of applications, including Fura-2 imaging – and this is particularly useful for imaging facilities.
For researchers with a dedicated microscope setup for Fura-2 applications, the pE-340fura is a three-channel LED Illumination System, which includes a broad spectrum white light LED in addition to the 340 nm and 380 nm LEDs.
It can still be used for exciting other indicators and fluorophores, but the white light does not offer the advanced spectral separation of the pE-800fura.
Which LED Illumination System for Fura-2?
Dr Martin Bootman explains his experience in his application note: Cell Biology in the Fast Lane: Analysing Fast Cellular Processes with LED Microscopy Illumination
Conclusion
In conclusion, powerful and controllable LED illumination has transformed widefield calcium imaging empowering researchers with efficiency and experimental flexibility. Its solid-state nature delivers high-speed switching and individual channel control, which are especially crucial for precise ratiometric measurements. As red-shifted dyes become more popular, LED illumination systems are also able to support this trend, promising a future of diverse applications.
Further Reading
A selection of peer-reviewed research papers utilising CoolLED Illumination Systems for a range of calcium indicators:
Fura-2
Skrabak D, Bischof H, Pham T, et al. Slack K+ channels limit kainic acid-induced seizure severity in mice by modulating neuronal excitability and firing. Commun Biol. 2023;6(1):1029. Published 2023 Oct 11. doi:10.1038/s42003-023-05387-9
Lia A, Sansevero G, Chiavegato A, et al. Rescue of astrocyte activity by the calcium sensor STIM1 restores long-term synaptic plasticity in female mice modelling Alzheimer’s disease. Nat Commun. 2023;14(1):1590. Published 2023 Mar 22. doi:10.1038/s41467-023-37240-2
Calcium Green
Zhuang X, Wong NF, Sun W, Xu-Friedman MA. Mechanisms and Functional Consequences of Presynaptic Homeostatic Plasticity at Auditory Nerve Synapses. J Neurosci. 2020;40(36):6896-6909. doi:10.1523/JNEUROSCI.1175-19.2020
Fluo-4
Okolo CA, Khaing EP, Mereacre V, et al. Direct regulation of the cardiac ryanodine receptor (RyR2) by O-GlcNAcylation. Cardiovasc Diabetol. 2023;22(1):276. Published 2023 Oct 13. doi:10.1186/s12933-023-02010-3
Trovato F, Stefani FR, Li J, et al. Transcription Factor-Forced Astrocytic Differentiation Impairs Human Glioblastoma Growth In Vitro and In Vivo. Mol Cancer Ther. 2023;22(2):274-286. doi:10.1158/1535-7163.MCT-21-0903
GCaMP
Taylor KR, Barron T, Hui A, et al. Glioma synapses recruit mechanisms of adaptive plasticity. Nature. 2023;623(7986):366-374. doi:10.1038/s41586-023-06678-1
Morris PG, Herbison AE. Mechanism of Arcuate Kisspeptin Neuron Synchronization in Acute Brain Slices From Female Mice. Endocrinology. 2023;164(12):bqad167. doi:10.1210/endocr/bqad167
Kondo T, Ebinuma I, Tanaka H, et al. Rapid and Robust Multi-Phenotypic Assay System for ALS Using Human iPS Cells with Mutations in Causative Genes. Int J Mol Sci. 2023;24(8):6987. Published 2023 Apr 10. doi:10.3390/ijms24086987
References:
- Lambert, TJ (2019) FPbase: a community-editable fluorescent protein database. Nature Methods.16, 277–278. doi: 1038/s41592-019-0352-8
- TINNING, P. W., FRANSSEN, A. J. P.M., HRIDI, S. U., BUSHELL, T. J. and MCCONNELL, G. (2017), A 340/380 nm light-emitting diode illuminator for Fura-2 AM ratiometric Ca2+imaging of live cells with better than 5 nM precision. Journal of Microscopy. doi:10.1111/jmi.12616